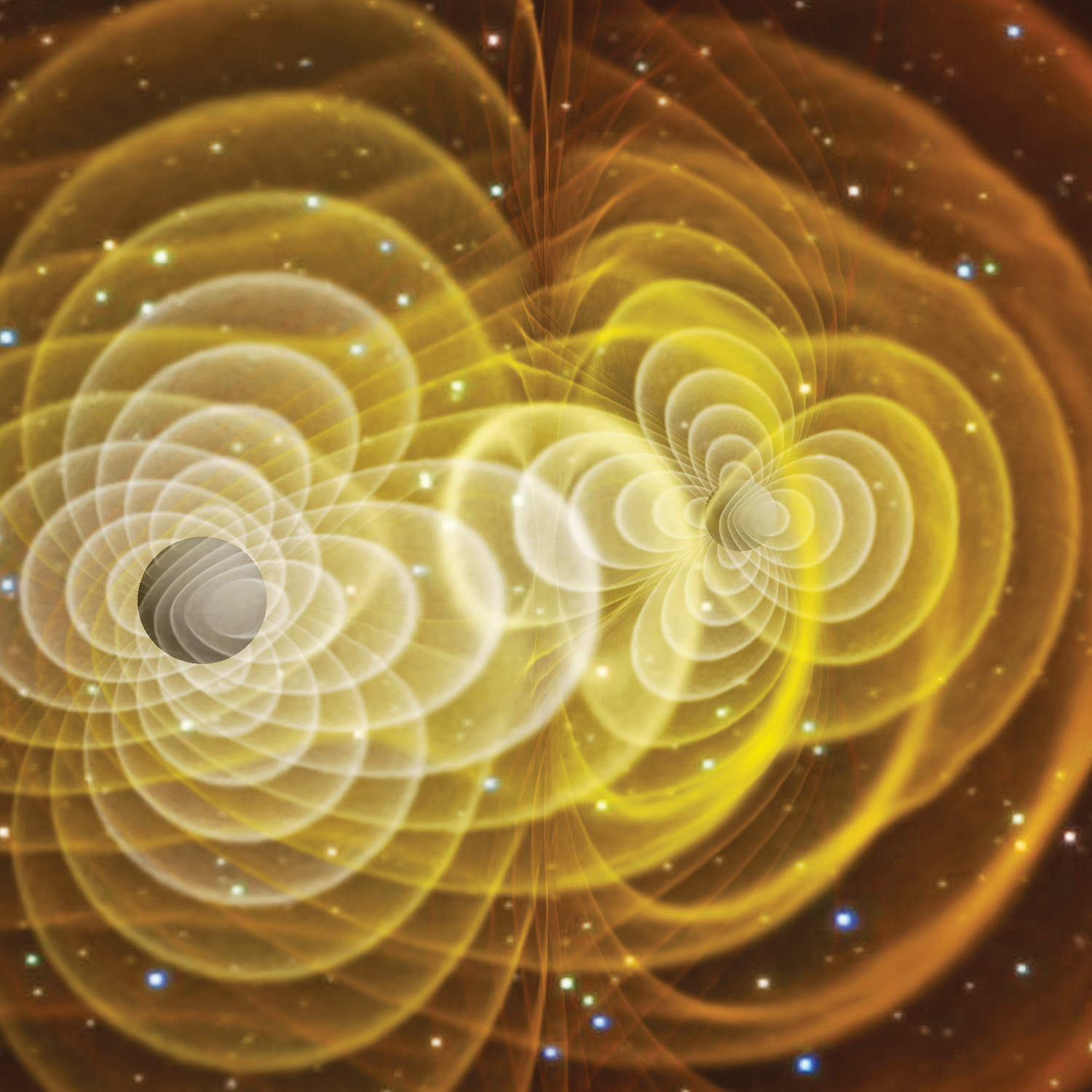
Monday 14 September 2015 was no ordinary day. At exactly 09:50:45 Universal Time, for one-fifth of a second, the Earth was stretched and squeezed by a tenth of a quintillionth of one per cent. Everything on the planet expanded and contracted as it did by one part in 1021 (1 followed by 21 noughts). It was proof that after a decades-long search, scientists had finally developed instruments sensitive enough to detect gravitational waves – ripples in space-time that were 10,000 times smaller than the nucleus of a hydrogen atom.
That at least begins to take care of when, where and what happened that Monday morning. In this engaging book the Dutch science writer Govert Schilling goes on to deal with the who and why by telling the tale of those involved in making what has been dubbed by some as “the discovery of the century” and the reason those unimaginably tiny ripples in space-time originated in a catastrophic event 1.3 billion years ago in a galaxy far, far away.
The “who” starts with a 36-year-old German physicist who in 1915 had just completed his masterwork, general relativity. In Albert Einstein’s new theory, gravity was due to the warping of space by the presence of mass. The Earth moves around the sun not because some mysterious invisible force pulls it, but because the warping of space tells matter how to move, while matter tells space how to curve. General relativity revealed that the familiar three-dimensions of space and the passage of time are not independent and absolute but are woven together into a four-dimensional fabric called space-time.
Einstein was fallible. Although vibrations in the fabric of space-time are a distinctive consequence of general relativity, Einstein wrote that “there are no gravitational waves”. He soon changed his mind; but the hunt for gravity waves using detectors in the lab would not begin until the late 1950s.
The Laser Interferometer Gravitational-wave Observatory, LIGO, was given the green light in 1990 by the US National Science Foundation, despite a $300 million price tag. By 2015 the project involved two similar detectors housed in facilities some 3,000 kilometres apart – one in Hanford, Washington State, the other in Livingston, Louisiana. A single detector would register microseismic events, such as passing cars; to exclude these false alarms, experimenters would take note only of events that showed up in both detectors within a few milliseconds of each other.
In the LIGO detectors, laser beams are fired along 4km-long L-shaped vacuum pipes and reflected from mirrors at each end. By analysing the light beams, it is possible to detect changes in the distance between the mirrors, which increases and decreases as space expands and contracts due to a passing gravitational wave. But the effect is tiny because gravity is a weak force and space-time is not easy to flex, bend, stretch or compress. A lot of energy is required for the tiniest ripples. Even pairs of stars orbiting each other don’t generate gravitational waves that LIGO can detect; but events involving black holes would.
Black holes, another prediction of general relativity, are the remnants of stars many times more massive than the sun. These stars burn brightly, and in their death throes, signalled by going supernova, their inner part collapses to form a black hole.
GW150914, the first gravitational wave detected by LIGO on 14 September 2015, was produced by the merger of two black holes that were 36 and 29 times as massive as the sun. As those two black holes orbited each other 1.3 billion years ago, they generated minute ripples in space-time that propagated with the speed of light. The waves carried away energy, causing the two holes to spiral ever closer, orbiting each other hundreds of times a second. As space-time was stretched and squeezed, the tiny perturbations grew into massive waves. When the two black holes collided and merged into one, a tsunami of gravitational waves was generated. These cataclysmic collisions happen less than once in a million years in our galaxy, but there are at least 100 billion galaxies in the observable universe.
“When I am judging a theory, I ask myself whether, if I were God, I would have arranged the world in such a way,” Einstein once confessed. Perhaps only he or Newton could get away with such a statement; the rest have to rely on the close relationship between theoretical insight and experimental scrutiny that lies at the heart of the scientific method. Wherever evidence can be coaxed out of nature, it corroborates or refutes a theory and serves as the sole arbiter of validity. Gravity waves are another tick for general relativity and the first direct proof of the existence of black holes; all other evidence has been circumstantial.
The hunt for gravity waves is over, but gravitational wave astronomy may help solve some mysteries that continue to baffle physicists: such as the nature of dark matter and dark energy, which together make up 96 per cent of the universe.
Ripples in Spacetime: Einstein, Gravitational Waves, and the Future of Astronomy
Govert Schilling
Harvard-Belknap, 340pp, £23.95
Manjit Kumar is the author of “Quantum: Einstein, Bohr and the Great Debate About the Nature of Reality” (Icon)
This article appears in the 16 Aug 2017 issue of the New Statesman, Trump goes nuclear